The case for warm water ocean fertilization
Why gigaton-scale carbon sequestration may work better in the tropics
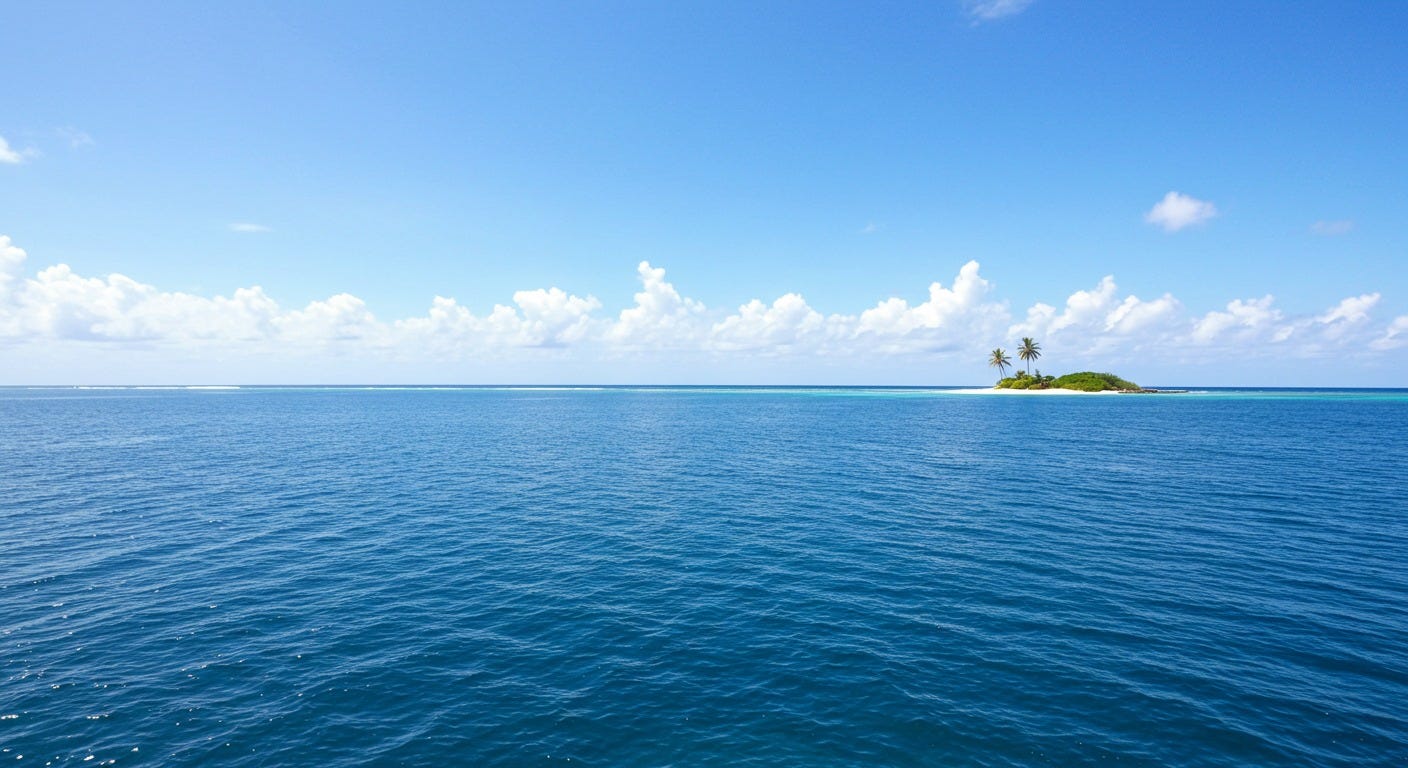
Scientists have been experimenting with ocean fertilization for decades. To date every single trial has been done in cold waters.
Places like the Gulf of Alaska and the vast Southern Ocean around Antarctica made ideal testing grounds for the hypothesis that got this whole field going: John Martin’s argument that it’s iron that limits the growth of many marine microorganisms.
Oceanographers call them High-Nutrient, Low-Chlorophyl (HNLC) waters": they have all the ingredients for photosynthesis except iron. It stood to reason that if you just added a bit of iron to those waters, you’d get a big boom in ocean life.
That’s now been shown to be true again and again and again.
But does that mean cold water is the best place to store carbon?
Not at all.
For decades scientists have pondered an alternative approach, focusing on warmer waters closer to the equator. These waters pose additional challenges, but they hold out the prospect for improved long-term carbon sequestration.
First, the challenge: warmer oceans are often low not just in iron but in nitrate, too. They’re Low-Nutrient, Low-Chlorophyll — LNLC.
That feels like it should be a deal-breaker, because phytoplankton need a lot more nitrate than iron: trace quantities won’t be enough.
In high-nutrient but iron-deficient waters, a single ton of iron is all you need to set off a bloom that captures ten thousand tons of carbon dioxide or more. But where macronutrients are low, the leverage ratios you get aren’t nearly so attractive.
For instance, adding a ton of nitrate will typically capture just five tons of carbon dioxide.
At first glance, that makes nitrate-deficient waters look like terrible candidates for fertilization. You’d think you’ll need a floating chemical fertilizer plant running a Haber-Bosch process to fix nitrogen from the air, some monstruosity that might look like:
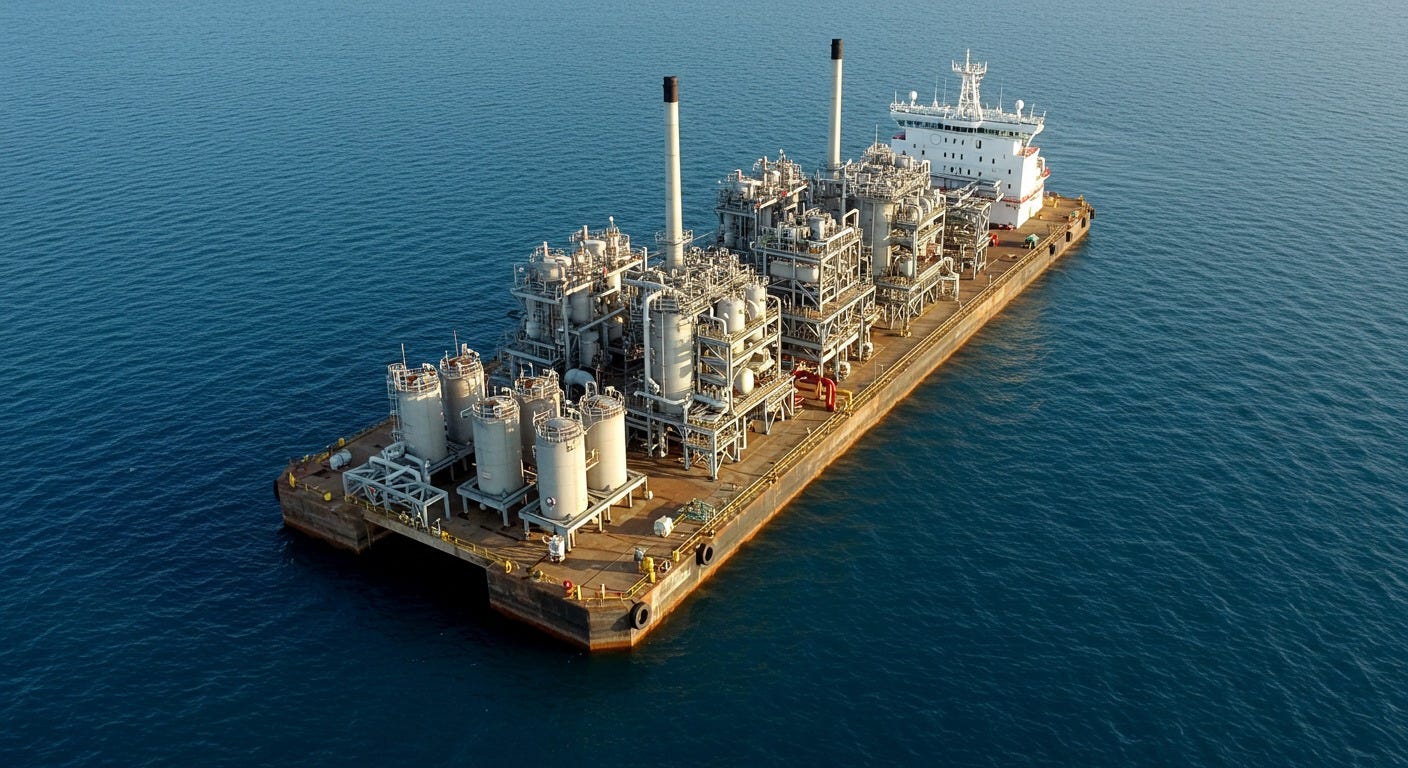
…which seems like a wildly impractical idea.
Except...what if you didn’t need a floating chemical plant to produce ammonium?
What if you could put bioavailable nitrogen in the water using some kind solar-power self-replicating nanobot?
That sounds way crazier, right?
Except nature had this idea millions of years before we did.
They’re called diazatrophs. They’re not bots, they’re marine microorganism. They’re already in the water. We just need to feed them.
Just like soils contain bacteria able to fix nitrogen from the air into a bioavailable form, oceans contain plenty of nitrogen fixing microorganisms that can do the same thing.
Diazatrophs use the same nitrogen-fixing enzyme as their cousins on land, from which they diverged evolutionarily some hundreds of millions of years ago. They’re in waters pretty much everywhere.
Which raises the question: well, what if you just stimulate them?
A new generation of researchers is considering an old idea to fertilize warm waters through a two-step process. Inspired by the naturally occurring fertilization in the Tonga Kermadec ridge, they think they can fertilize subtropical oceans by fertilizing the diazatrophs that fix the nitrogen that fertilizes everything else.
Why would you go to all that trouble? Because the same ocean dynamics that make warm waters nutrient-poor also make them much more conducive to long-term carbon sequestration.
We know cold waters are rich in nutrients. Why? Because they get brought up from the deep ocean, where nutrient concentrations are higher. Near the poles, strong winds continually mix surface and deep waters. There’s not much sunlight to warm these waters once they reach the surface, so they don’t stratify in the same way they would in tropical oceans. The result is continual vigorous mixing between surface waters and deep waters: as soon as nutrients are depleted from surface waters they are replenished by mixing of new nutrient-rich waters from the deep.
That’s why nutrient-rich cold water fertilization is excellent at generating big phytoplankton blooms, but also pretty poor at storing carbon for a long time. The same churning that brings up the nutrient also brings up the carbon stored deep down. Once it’s in the surface layer, it can easily re-emerge into the atmosphere again.
In warm waters you get the opposite dynamic. These oceans tend to see a lot less vertical mixing. The sun warms the top layer. Warm water is less dense, and more buoyant, so that warmer top layer stays parked on top of the water column. Lower down, more nutrient-dense layers stay cold, and therefore dense, and also away from the sun. That’s stratification: the warm water on top is much less dense than the cold layers below, so the two don’t mix. So whatever sinks down tends to stay down including both nutrients like and also carbon.
You start to see why this is interesting for carbon sequestration. If you can get big phytoplankton blooms in warm waters, you multiply the chances of long-term storage. Particulate carbon that sinks down through warm water has a much higher chance of staying down.
Of course, most phytoplankton won’t sink down. Most of it will be eaten by bigger organisms. As those grazers turn phytoplankton into chemical energy, its components will be respired back out into the water. Here comes the neat part: when that happens, the carbon is reinjected into the water, but so are the nitrates. Those nutrients remain near the surface, where new phytoplankton can use them to photosynthesize.
That cycling can continue indefinitely until, eventually, a given photosynthesizer just happens to escape all predators as he makes his way down the water column, sequestering its carbon content for hundreds or thousands of years.
This is what makes warm water fertilization so interesting. With each cycle, it’s like we’re buying a lottery ticket for each individual photosynthetic organism. On any given cycle, the chances that any individual wins the lottery (prize: a one way ticket down to the deep ocean) is low. But repeat that again and again and the chances of a given carbon molecule getting sequestered over the long term converges to 1.
This, at any rate, is the theory.
We really should test it. If it pans out, it could make warm water fertilization a stand-out star among proposed techniques for marine Carbon Dioxide Removal: a low cost, ecologically benign mechanism that could be scaled up across the world’s massive subtropical ocean gyres.
Of course, it may not pan out.
It may be that the people working on cold water fertilization figure out ways of sidestepping the problems outlined above, and it ends up being that they solve the problem first.
And it could also be the case that both systems end up working in parallel. I don’t know.
What I know for sure is that we won’t be able to tell until we try it.
Hmmm. Sounds like wave-driven upwelling pumps deliver the goods, 24/7/365. Upwelling the full suite of nutrients, triggering photosynthesis, increasing pH, with primary producers feeding the fish which poop the carbon. Theen there are salps......and many other beneficial critters.
HABs will be more prevalent in warm waters and more difficult to control if mitigatable at all. Yes, the kinetics are stronger in the northern latitudes and even stronger in the southern ocean, but the NE Pacific (Gulf of Alaska) has had a fair amount of stratification, SST problems over the past 15 years. I suspect the thermocline is stronger in warm equatorial surface waters (maybe winter in warm waters would be optimal).